Registration Dossier
Registration Dossier
Data platform availability banner - registered substances factsheets
Please be aware that this old REACH registration data factsheet is no longer maintained; it remains frozen as of 19th May 2023.
The new ECHA CHEM database has been released by ECHA, and it now contains all REACH registration data. There are more details on the transition of ECHA's published data to ECHA CHEM here.
Diss Factsheets
Use of this information is subject to copyright laws and may require the permission of the owner of the information, as described in the ECHA Legal Notice.
EC number: 701-133-3 | CAS number: -
- Life Cycle description
- Uses advised against
- Endpoint summary
- Appearance / physical state / colour
- Melting point / freezing point
- Boiling point
- Density
- Particle size distribution (Granulometry)
- Vapour pressure
- Partition coefficient
- Water solubility
- Solubility in organic solvents / fat solubility
- Surface tension
- Flash point
- Auto flammability
- Flammability
- Explosiveness
- Oxidising properties
- Oxidation reduction potential
- Stability in organic solvents and identity of relevant degradation products
- Storage stability and reactivity towards container material
- Stability: thermal, sunlight, metals
- pH
- Dissociation constant
- Viscosity
- Additional physico-chemical information
- Additional physico-chemical properties of nanomaterials
- Nanomaterial agglomeration / aggregation
- Nanomaterial crystalline phase
- Nanomaterial crystallite and grain size
- Nanomaterial aspect ratio / shape
- Nanomaterial specific surface area
- Nanomaterial Zeta potential
- Nanomaterial surface chemistry
- Nanomaterial dustiness
- Nanomaterial porosity
- Nanomaterial pour density
- Nanomaterial photocatalytic activity
- Nanomaterial radical formation potential
- Nanomaterial catalytic activity
- Endpoint summary
- Stability
- Biodegradation
- Bioaccumulation
- Transport and distribution
- Environmental data
- Additional information on environmental fate and behaviour
- Ecotoxicological Summary
- Aquatic toxicity
- Endpoint summary
- Short-term toxicity to fish
- Long-term toxicity to fish
- Short-term toxicity to aquatic invertebrates
- Long-term toxicity to aquatic invertebrates
- Toxicity to aquatic algae and cyanobacteria
- Toxicity to aquatic plants other than algae
- Toxicity to microorganisms
- Endocrine disrupter testing in aquatic vertebrates – in vivo
- Toxicity to other aquatic organisms
- Sediment toxicity
- Terrestrial toxicity
- Biological effects monitoring
- Biotransformation and kinetics
- Additional ecotoxological information
- Toxicological Summary
- Toxicokinetics, metabolism and distribution
- Acute Toxicity
- Irritation / corrosion
- Sensitisation
- Repeated dose toxicity
- Genetic toxicity
- Carcinogenicity
- Toxicity to reproduction
- Specific investigations
- Exposure related observations in humans
- Toxic effects on livestock and pets
- Additional toxicological data
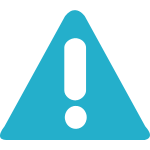
Basic toxicokinetics
Some information in this page has been claimed confidential.
Administrative data
- Endpoint:
- basic toxicokinetics, other
- Type of information:
- (Q)SAR
- Adequacy of study:
- key study
- Study period:
- 2019
- Reliability:
- 2 (reliable with restrictions)
- Rationale for reliability incl. deficiencies:
- accepted calculation method
Data source
Reference
- Reference Type:
- study report
- Title:
- Unnamed
- Year:
- 2 019
- Report date:
- 2019
Materials and methods
- Objective of study:
- metabolism
- toxicokinetics
- Principles of method if other than guideline:
- - Software tool(s) used including version:
OASIS TIMES platform for simulating metabolism
OECD QSAR Toolbox
- Model description: see field 'Justification for non-standard information', 'Attached justification' and/or 'Cross-reference'
- Justification of QSAR prediction: see field 'Justification for type of information', 'Attached justification' and/or 'Cross-reference'
- Details on the 3 TIMES simulators:
1- in vitro liver S9 metabolic simulator
The in vitro rodent microsomal/S9 mix metabolic simulator reproduces and predicts the metabolic pathways of xenobiotic chemicals for in vitro experimental systems such as rodent (mostly rat) liver microsomes and S9 fraction. The current in vitro rat liver metabolic simulator represents electronically designed set of 517 structurally generalized, hierarchically arranged biotransformation reactions. The metabolism training set contains experimentally documented in vitro metabolic pathways for 261 parent chemicals of a wide structural diversity, and 978 observed metabolites compiled into a searchable electronic database.
As configured in the TIMES system, options for generation of metabolic maps are set to reproduce no more than three levels or confined by products probability threshold (“weight” ≥ 0.1). This is a practical limitation for confining propagation of simulated metabolism maps based on observation that the documented metabolism usually ends up at third transformation level. Also, the limitation is related with the fact that metabolites generated up to the third level are enough to justify the prediction for in vitro mutagenicity endpoints.
2- In vivo rat (whole organisms) metabolic simulator
The in vivo rodent metabolic simulator reproduces and predicts the metabolic pathways of xenobiotic chemicals in vivo in rodents (mostly rats). The metabolism training set contains experimentally documented in vivo metabolic pathways for 647 structurally different parent chemicals, and 4382 observed metabolites compiled into a searchable electronic database. The current in vivo rat metabolic simulator represents electronically designed set of 622 structurally generalized, hierarchically arranged biotransformation reactions. These molecular transformations describe in vivo metabolism in rats accounting for the whole organism.
As configured in the TIMES system, propagation of in vivo metabolism is confined by a threshold of probabilities to generate metabolites (i.e., probabilities to produce metabolites to be ≥ 0.02).
3- Skin metabolic simulator
The skin metabolism simulator reproduced documented in vitro metabolism of 183 unique parent chemicals having 206 documented metabolic maps. Although in vitro data are used to build this metabolism simulator, given the absence of in vivo metabolism data it is assumed that in vitro data should allow adequately prediction of in vivo metabolism in skin. The current metabolic simulator represents electronically designed set of 276 structurally generalized, hierarchically arranged biotransformation reactions. These molecular transformations describe the metabolism of chemicals in the skin compartment and further used to simulate the enzymatic activation of chemicals when predict skin sensitization.
As configured in the TIMES system, options for generation of metabolic maps are set to reproduce no more than four levels of metabolism or confined by using threshold for probability of generated metabolites (“weight” ≥ 0.003). These limitations are related with the fact that the most probable metabolites associated with skin sensitization effect are generated up to the fourth level of metabolism. - GLP compliance:
- no
Test material
- Details on test material:
- - Name of test material (as cited in study report): 3,5,5 trimethylhexyl 3,5,5 trimethylhexanoate
- Physical state: clear liquid
- Analytical purity: 100%
Constituent 1
- Specific details on test material used for the study:
- The QSAR approah had considered and compared the metabolism of the monoconstituent 3,5,5-trimethylhexyl 3,5,5-trimethylhexanoate (RN CAS 59219-71-5), and of the UVCB branched-nonyl 3,5,5- trimethylhexanoate.
Results and discussion
Metabolite characterisation studies
- Metabolites identified:
- yes
Any other information on results incl. tables
SMILES of 3,5,5 trimethylhexyl 3,5,5 trimethylhexanoate (RN CAS 59219-71-5) (monoconstituent) and SMILES of the representative constituents, with the monoconstituent, of branched 3,5,5- trimethylhexanoate (UVCB) were used.
1. Metabolism of target chemicals
No documented metabolismof the target chemicals has been found in the scientific literature.
2. Metabolism of structural analogues
Exhaustive search in the literature has been performed to identify analogues of the target chemicals having documented metabolism in in vitro S9, in vivo (whole organism) or skin. As a result, four analogues have been found only being common with the target chemicals with respect to the ester functionality. Structural similarity of the analogues has been ignored to some extent at the cost of the preserving ester functionality which, as shown further, determine the principle molecular transformations of target chemicals. In other words, structural analogues of the target chemicals having documented metabolism have been used to confirm that the main metabolic transformation is ester hydrolysis.
Documented metabolism of these four aliphatic alkyl esters which are analogues of the target chemicals with respect to the ester functionality has been found in:
Ø In vitro rat liver S9 (one analogue);
Ø In vivo rat (three analogues);
Ø Skin metabolism (one analogue).
The structural analogues with information for availability of their documented metabolism are presented in Table 2:
Table 2.Documentedmetabolismof structural analogues.
## |
Analogues |
In vitro ratliver S9 documented metabolism |
In vivodocumented metabolism |
Skin documented metabolism |
1 |
CAS 540-88-5 tert-butyl acetate |
N/A |
Yes [6] |
N/A |
2 |
CAS 103-23-1 Bis (2-ethylhexyl) adipate |
Yes [7] |
Yes [7] |
N/A |
3 |
CAS 123-86-4 butyl acetate |
N/A |
Yes [8] |
N/A |
4 |
CAS 14062-23-8 4-Biphenylacetic acid, ethyl ester |
N/A |
N/A |
Yes [9] |
4-Biphenylacetic acid, ethyl ester (the last analogue in Table 2) is the most dissimilar analogue having commonality to the target compounds only with respect to the presence of an ester group. We have used it in the current analysis, because this is the only aliphatic acid ester analogue for which documented metabolism in skin has been found in literature.
In vitro rat liver S9 metabolism
Simulated in vitro rat liver S9 metabolic map of the structural analogue bis(2 -ethylhexyl)adipate (CAS: 103 -23 -1). The major pathway is ester hydrolysis which is obtained with very high probability (P > 0.9). Ester hydrolysis leads to the formation of two metabolites–alcohol and acid. The mono- and dicarboxylic acids (highlighted in green) are documented and simulated correctly by TIMES. It is important to mention that not all simulated metabolites are documented. However, it does not mean that these metabolites are not formed in reality – they are just not documented. Their adequacy is usually analyzed by evaluation of the simulated metabolic “maps” by third-party experts
§ Documented in vitro metabolites are simulated correctly.
§ The major metabolic pathway is enzymatic ester hydrolysis,which results in the formation of acid and alcohol metabolites.
§ Simulated are some additional oxidation reactions - the secondary hydrolysis product could be oxidized via aldehyde to the corresponding acid.
§ No Phase II metabolites are generated up to three levels of simulation
In vivo rat (whole organism) metabolism
Simulated in vivo metabolic maps of three structural analogues are presented in this section.
Analogue # 1 -Chemical ID:Name:tert-butylacetate; CAS # 540-88-5
Analogue # 2 -Chemical ID: Name: bis(2-ethylhexyl) adipate; CAS # 103-23-1
Analogue # 3 -Chemical ID:Name: butyl acetate; CAS # 123-86-4
§ Documented in vivo metabolites are simulated correctly.
§ The major simulated metabolic transformation is ester hydrolysis which occurs as a first step (with high probability, P > 0.9) and leads to the formation of acid and alcohol.
§ The alcohol metabolite with a primary alcohol group is further oxidized via aldehyde intermediate to the corresponding carboxylic acid.
§ Significant amount ofPhase II metabolites is obtained in vivo as compared with in vitro metabolism.
Skin metabolism
Documented skin metabolism has been found for one structural analogue (4-Biphenylacetic acid, ethyl ester, CAS # 14062-23-8).
The selected analogue (4-Biphenylacetic acid, ethyl ester) having benzyl-type biphenyl fragment next to the alkyl ester functionality is structurally dissimilar to some extent to the targets. Still, ester hydrolysis occurs as primary metabolic transformation forming an acid and alcohol in skin. This metabolic reaction is more facilitated in the presence of benzyl-type biphenyl fragment due to its slight electron-withdrawing effect. This could increase the partial positive charge on the carbonyl carbon atom and the reactivity of the ester carboxyl group towards the SN2-type hydrolysis.
It should be stressed that carboxylesterase enzymatic system which is responsible for the ester hydrolysis in liver is also expressed in skin
Significant amount of Phase II metabolites is obtained by the skin metabolism simulator. However, one should emphasize that the specific enzymatic activity of Phase II UDP-glucuronosyltransferase in skin is reported to be about 10 – 50 % of that in rat liver microsomes. On the other hand, the same UDP-glucuronosyltransferase is significantly more expressed in vivo than in in vitro S9 due to the fact that the whole organism in vivo is multi-organ system involving not only liver but also bile, GI tract, etc. Hence, Phase II glucuronides of a given substrate should be essentially more abundant in vivo followed by in vitro microsomal/S9 system and, ultimately, skin. Nevertheless, the products of esters hydrolysis in skin – acid and alcohol are directly involved in Phase II metabolic reactions, instead of undergoing Phase I aliphatic C-oxidations which occur with the in vitro microsomal/S9 and in vivo.This could be justified by the fact that Phase I oxidizing enzymes such as cytochromes (CYPs), alcohol/aldehyde dehydrogenase, aldehyde oxidase, etc. show lower specific activities in skin than in microsomes and liver. This explains why oxidative reactions such as aliphatic C-oxidations, occurring mainly in liver and following ester hydrolysis are more pronounced in vivo than in skin. The lower activities of Phase I oxidizing enzymes in skin causes an increase in the relative role of Phase II conjugation reactions, although Phase II enzymatic activities in skin are lower as compared to in vivo and in vitro S9 systems.
Similar result is obtained by the TIMES in vivo metabolic simulator.
Conclusions:
§ Documented metabolites are simulated correctly by TIMES.
§ Same enzymes controlling metabolism inin vivorat are involved in the metabolism in skin. However, activity of these enzymes is different in all three metabolic systems which provides some specificity of skin metabolism:
Activity of Phase I oxidizing enzymes, such as alcohol/aldehyde dehydrogenase and aldehyde oxidase, is lower in skin as compared to S9 and liver.
Lower activity of Phase I oxidizing enzymes increases the relative importance of Phase II metabolic reactions (such as glucuronidation) in skin which, otherwise, are less expressed in skin as compared to in vivo and in vitro S9.
§
§ Similarity between skin andin vivo rat metabolism has been found with respect to simulation of Phase II metabolites. Both simulators provide large amount of Phase II metabolites.
§ The major simulated metabolic transformation is enzymatic ester hydrolysis which occurs with probability P > 0.9 and results in the formation of acid and alcohol.
General conclusions on simulated metabolism of the structural analogues:
A. Documented metabolites are simulated correctly by all three (in vitro S9 mix, in vivo whole organism and skin) TIMES metabolic simulators. The correctly simulated ester hydrolysis pathway is a premise for adequate model predictions.
B. Same pattern is obtained by all three TIMES metabolic simulators:
a) Ester hydrolysis is first and major transformation obtained with high probability (P > 0.9).
b) Primary alcohol is documented and simulated. This product of ester hydrolysis is further oxidized via aldehyde intermediate to carboxylic acid.
C. Activity of Phase II enzymes such as UDP-glucuronosyltranferase is more pronounced for:
a) In vitro microsomal/S9 system as compared to skin;
b) In vivo (whole organism) as compared to in vitro.
D. However, the relative amount of Phase II metabolites is higher in skin as compared with S9 and in vivo at the cost of the lower Phase I (oxidative) enzymatic activities.
E. Phase II metabolic transformations are acting as detoxification reactions in vivo by increasing the water solubility (hydrophilicity) of the substrates, and facilitating their renal and biliary excretion via the resulting Phase II conjugates
3. Simulating metabolism of the target chemicals
In vitro rat liver S9 metabolism
All target chemicals, considered as parent compounds, belong 100% to the parametric and above 80% to the structural domain. Hence, the prediction could be considered as reliable at domain threshold > 80%.
All target chemicals have same metabolic pattern which is similar to the S9 metabolic pattern of the analogue:
· The first and major metabolic transformation is enzymaticester hydrolysisoccurring with very high probability (P > 0.9);
· Alcohol product is further oxidized via aldehyde intermediates to corresponding carboxylic acids;
· The other metabolic product - carboxylic acid undergoes directPhase IIglucuronidation.
· Predictions could be considered as reliable at domain threshold above 80%.
In vivo (whole organisms) metabolism
All target chemicals belong 100% to the parametric and above 75% to the structural domain. Hence, the prediction could be considered as reliable at domain threshold > 75%.
in vivo ester hydrolysis leads to the formation of two metabolic products–alcohol and acid. The alcohol is further oxidized via aldehyde intermediate to the corresponding carboxylicacids. This metabolic pathway is common for both in vitro and in vivo metabolism of this metabolic product. However, as light difference with respect to the second metabolic product (the acid) has been found in in vivo,as reported in literature. Concisely, the acid metabolite contains a methyl substituent at β-position which blocks certain steps in the β-oxidation,typical for straight-chain fatty carboxylic acids in vivo. Alternatively, ω-hydroxylation at the terminal C{sp3}carbon atom is more plausible metabolic pathway as shown for some saturated, branched chain carboxylic acids. Example for such ω-hydroxylation reaction is provided for Phytanicacid (CAS: 14721-66-5) as illustrated below.
Figure. Metabolic ω-hydroxylation of Phytanic acid
ω-Hydroxylation can be further followed by oxidation of the primary alcohol to aldehyde, which is then oxidized to the corresponding carboxylic acid. Ultimately, dicarboxylic acids might be formed.
Hence, the ω-hydroxylation of acids, as ester hydrolysis products, is a slightly different in vivo metabolic pathway as compared to in vitro S9 rat, where the corresponding acid is directly involved in Phase II glucuronidation. Analogically, in skin metabolism (see next section) the acid obtained by the ester hydrolysis is also involved in Phase II reactions.
Conclusions:
Same in vivo metabolic pattern is obtained for the four target chemicals:
• Ester hydrolysis – first and major metabolic transformation with very high probability (P > 0.9);
• Two products of ester hydrolysis are obtained – alcohol and acid. Alcohol is further oxidized to corresponding carboxylic acids, whereas acid undergoes hydroxylation at the terminal (ω) carbon, ultimately leading to Phase II conjugates.
• Phase II reactions are more pronounced in vivo than in in vitro S9.
• Prediction could be considered as reliable at domain threshold >75%.
Skin metabolism
The predictions are reliable given the fact that all target chemicals belong 100% to parametric and structural domain.
Conclusions:
• Simulated skin metabolism is similar to the metabolism obtained by the in vitro S9 and in vivo mammalian metabolic simulators:
• Ester hydrolysis is the first and major metabolic transformation which occurs with very high probability (P > 0.9).
• Both ester hydrolysis products further undergo Phase II conjugation reactions.
• Same enzymes that control in vitro S9 and in vivo (whole organism) metabolism are involved in skin metabolism with activity : in vivo > in vitro S9 > skin. The corresponding differences in enzymes activity provide specificity of the skin metabolism:
• Phase I oxidizing enzymes such as alcohol/aldehyde dehydrogenase and aldehyde oxidase show lower activity in skin as compared to liver.
• Lower activity of Phase I enzymes in skin increases the relative amount of Phase II metabolites as compared to in vivo (whole organism) and in vitro S9 metabolism.
• The products (an acid and alcohol) of enzymatic hydrolysis in skin undergo direct Phase II metabolic reactions rather than Phase I aliphatic C-oxidation as in in vitro S9 and in vivo metabolism.
• Kinetics of biotransformations are not provided in the current TIMES metabolic simulators reproducing metabolic transformation statically – within the time frame of the experimental conditions.
• The predictions are considered as reliable as all target chemicals belong 100% to parametric and structural domain.
Applicant's summary and conclusion
- Conclusions:
- A. No documented metabolism of the target chemicals has been found.
B. Structural analogues of the target chemicals having documented metabolism have been used to confirm the main metabolic transformation - ester hydrolysis.
C. No difference between simulated metabolism pattern of the target chemicals within each of the analyzed (in vivo, in vitro S9, skin) metabolic systems.
D. Commonality of simulated metabolism of the target chemicals with respect to all three metabolic systems is the first and major metabolic transformation, enzymatic ester hydrolysis, which occurs with very high probability (P > 0.9).
E. Metabolic pattern of the acid hydrolysis product (left branch in the maps) in all three metabolic systems could be summarized as follows:
a. Similar metabolism has been found in in vitro microsomes/S9 and skin, where the acid is directly involved in Phase II conjugation reactions.
b. Slightly different transformation has been found for in vivo metabolism, where the acid undergoes hydroxylation at the terminal (ω) carbon, ultimately leading to Phase II conjugates.
F. Metabolism of the alcohol hydrolysis product (right branch in the maps) in all three metabolic systems could be summarized as follows:
a. Similarity has been found for in vitro microsomes/S9 and in vivo metabolism. The only difference is an additional Phase II glucuronidation reaction in vivo which does not occur in vitro S9.
b. Slightly different transformation has been found in skin metabolism due to the lower activity of Phase I oxidizing enzymes as compared to in vitro S9 and in vivo systems. Lower activity of these oxidizing enzymes in skin increases the relative importance of Phase II metabolic reactions.
G. Kinetics of biotransformations are not provided in the current TIMES metabolic simulators reproducing metabolic transformation statically – within the time frame of the experimental conditions. Nevertheless, the different activity of enzymes in the three metabolic systems is not expected to affect significantly the rate of metabolic reactions.
H. According to our experts, no alerts associated with skin sensitization and genotoxicity could be expected in the target chemicals and the obtained metabolites.
I. All predictions could be considered as reliable given the fact that all target chemicals belong 100% to the parametric domain and above 75% to the structural domain. - Executive summary:
The QSAR Approach developped by the Laboratory of Mathematical Chemistry, Bourgas, Bulgaria, consist in simulating metabolism of aliphatic alkyl esters by TIMES.
4 combined elements were used :
• OASIS TIMES platform for simulating metabolism; with TIMES metabolic simulators used:
• in vitro rat liver S9;
• in vivo rat whole organisms;
• Skin metabolism.
• Toolbox 4.2 for searching analogues of the target chemicals;
• Documented metabolism from research publications and websites;
• Expert evaluation of the simulated metabolism.
SMILES of target substances were used as input for the model : SMILES of 3,5,5 trimethylhexyl 3,5,5 trimethylhexanoate (RN CAS 59219-71-5) (monoconstituent) and SMILES of the representative constituents, with the monoconstituent, of branched 3,5,5- trimethylhexanoate (UVCB)
3 Simulators were considered :
In vitro rat liver S9 (one analogue);
In vivo rat (three analogues);
Skin metabolism (one analogue).
Documented metabolites are simulated correctly by all three (in vitro S9 mix, in vivo whole organism and skin) TIMES metabolic simulators. The correctly simulated ester hydrolysis pathway is a premise for adequate model predictions.
Same pattern is obtained by all three TIMES metabolic simulators:
a) Ester hydrolysis is first and major transformation obtained with high probability (P > 0.9).
b) Primary alcohol is documented and simulated. This product of ester hydrolysis is further oxidized via aldehyde intermediate to carboxylic acid.
Formation of Phase II metabolites is more pronounced in vivo and in skin than in in vitro S9 metabolism.
For the simulating metabolism of the target chemicals, following results were obtained:
A. No documented metabolism of the target chemicals has been found.
B. Structural analogues of the target chemicals having documented metabolism have been used to confirm the main metabolic transformation - ester hydrolysis.
C. No difference between simulated metabolism pattern of the target chemicals within each of the analyzed (in vivo, in vitro S9, skin) metabolic systems.
D. Commonality of simulated metabolism of the target chemicals with respect to all three metabolic systems is the first and major metabolic transformation, enzymatic ester hydrolysis, which occurs with very high probability (P > 0.9).
E. Metabolic pattern of the acid hydrolysis product (left branch in the maps) in all three metabolic systems could be summarized as follows:
a. Similar metabolism has been found in in vitro microsomes/S9 and skin, where the acid is directly involved in Phase II conjugation reactions.
b. Slightly different transformation has been found for in vivo metabolism, where the acid undergoes hydroxylation at the terminal (ω) carbon, ultimately leading to Phase II conjugates.
F. Metabolism of the alcohol hydrolysis product (right branch in the maps) in all three metabolic systems could be summarized as follows:
a. Similarity has been found for in vitro microsomes/S9 and in vivo metabolism. The only difference is an additional Phase II glucuronidation reaction in vivo which does not occur in vitro S9.
b. Slightly different transformation has been found in skin metabolism due to the lower activity of Phase I oxidizing enzymes as compared to in vitro S9 and in vivo systems. Lower activity of these oxidizing enzymes in skin increases the relative importance of Phase II metabolic reactions.
G. Kinetics of biotransformations are not provided in the current TIMES metabolic simulators reproducing metabolic transformation statically – within the time frame of the experimental conditions. Nevertheless, the different activity of enzymes in the three metabolic systems is not expected to affect significantly the rate of metabolic reactions.
H. According to our experts, no alerts associated with skin sensitization and genotoxicity could be expected in the target chemicals and the obtained metabolites.
I. All predictions could be considered as reliable given the fact that all target chemicals belong 100% to the parametric domain and above 75% to the structural domain.
Information on Registered Substances comes from registration dossiers which have been assigned a registration number. The assignment of a registration number does however not guarantee that the information in the dossier is correct or that the dossier is compliant with Regulation (EC) No 1907/2006 (the REACH Regulation). This information has not been reviewed or verified by the Agency or any other authority. The content is subject to change without prior notice.
Reproduction or further distribution of this information may be subject to copyright protection. Use of the information without obtaining the permission from the owner(s) of the respective information might violate the rights of the owner.

Welcome to the ECHA website. This site is not fully supported in Internet Explorer 7 (and earlier versions). Please upgrade your Internet Explorer to a newer version.
This website uses cookies to ensure you get the best experience on our websites.
Find out more on how we use cookies.