Registration Dossier
Registration Dossier
Data platform availability banner - registered substances factsheets
Please be aware that this old REACH registration data factsheet is no longer maintained; it remains frozen as of 19th May 2023.
The new ECHA CHEM database has been released by ECHA, and it now contains all REACH registration data. There are more details on the transition of ECHA's published data to ECHA CHEM here.
Diss Factsheets
Use of this information is subject to copyright laws and may require the permission of the owner of the information, as described in the ECHA Legal Notice.
EC number: - | CAS number: -
- Life Cycle description
- Uses advised against
- Endpoint summary
- Appearance / physical state / colour
- Melting point / freezing point
- Boiling point
- Density
- Particle size distribution (Granulometry)
- Vapour pressure
- Partition coefficient
- Water solubility
- Solubility in organic solvents / fat solubility
- Surface tension
- Flash point
- Auto flammability
- Flammability
- Explosiveness
- Oxidising properties
- Oxidation reduction potential
- Stability in organic solvents and identity of relevant degradation products
- Storage stability and reactivity towards container material
- Stability: thermal, sunlight, metals
- pH
- Dissociation constant
- Viscosity
- Additional physico-chemical information
- Additional physico-chemical properties of nanomaterials
- Nanomaterial agglomeration / aggregation
- Nanomaterial crystalline phase
- Nanomaterial crystallite and grain size
- Nanomaterial aspect ratio / shape
- Nanomaterial specific surface area
- Nanomaterial Zeta potential
- Nanomaterial surface chemistry
- Nanomaterial dustiness
- Nanomaterial porosity
- Nanomaterial pour density
- Nanomaterial photocatalytic activity
- Nanomaterial radical formation potential
- Nanomaterial catalytic activity
- Endpoint summary
- Stability
- Biodegradation
- Bioaccumulation
- Transport and distribution
- Environmental data
- Additional information on environmental fate and behaviour
- Ecotoxicological Summary
- Aquatic toxicity
- Endpoint summary
- Short-term toxicity to fish
- Long-term toxicity to fish
- Short-term toxicity to aquatic invertebrates
- Long-term toxicity to aquatic invertebrates
- Toxicity to aquatic algae and cyanobacteria
- Toxicity to aquatic plants other than algae
- Toxicity to microorganisms
- Endocrine disrupter testing in aquatic vertebrates – in vivo
- Toxicity to other aquatic organisms
- Sediment toxicity
- Terrestrial toxicity
- Biological effects monitoring
- Biotransformation and kinetics
- Additional ecotoxological information
- Toxicological Summary
- Toxicokinetics, metabolism and distribution
- Acute Toxicity
- Irritation / corrosion
- Sensitisation
- Repeated dose toxicity
- Genetic toxicity
- Carcinogenicity
- Toxicity to reproduction
- Specific investigations
- Exposure related observations in humans
- Toxic effects on livestock and pets
- Additional toxicological data
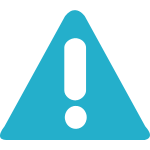
Endpoint summary
Administrative data
Key value for chemical safety assessment
Effects on fertility
Additional information
No effects were observed on reproductive organs or tissues in an extended OECD422 study, therefore performing further studies is not required.
Short description of key information:
Reproduction was unaffected up to 2000 mg/kg body weight/day in an extended OECD422 screening study.
Effects on developmental toxicity
Description of key information
Development was unaffected up to 2000 mg/kg body weight/day in an extended OECD422 screening study. Performing a developmental toxicity study is scientifically unjustified due to the fact that there is data on the different components of the “Complexation products of sodium tartrate with iron trichloride” that indicates there is no hazard.
Additional information
Performing a developmental toxicity study is scientifically unjustified due to the fact that there is data on the different components of the “Complexation products of sodium tartrate with iron trichloride” that indicates there is no hazard. For details see the text below.
Tartaric acid
At its meeting in 1977, JECFA summarized the outcome of teratology studies conducted in rats, mice, hamsters, and rabbits. In these studies, tartaric acid administered during organogenesis did not result in maternal toxicity or adverse effects on pup survival or soft/skeletal tissues at in various laboratory animal species. The same data were also considered during the assessment of tartaric acid as a GRAS food ingredient. Although these studies were unpublished, they are presented below as supporting documentation of the safety of tartaric acid (FDRL, 1973).
In the first study, adult female albino mice were mated with young male mice. Food and water was provided ad libitum. Administration of the test compounds began on the 6th day of the gestation period. Group of 20 to 23 female mice were administered either a sham dose (negative control), 150 mg/kg body weight/day of aspirin (positive control), or 2.74, 12.7, 59.1, or 274.0 mg tartaric acid/kg body weight/day in a volume of 1 mL/kg body weight. The administration was continued through Day 15 of gestation. During gestation, the dams were examined daily and the food and water consumption were measured. Body weight was measured on Days 0, 6, 11, 15, and 17 of gestation. On the 17th day of gestation, the pups were delivered via caesarean section and the pups were examined. Parameters measured included the number of implantation sites, resorption sites, live foetuses, and dead foetuses. The body weights of live foetuses were recorded and all foetuses were subject to an examination of external abnormalities. The foetuses of each litter were then subdivided with one-third receiving a detailed visceral examination according to the Wilson method (Wilson, 1965) and the remaining two-thirds dyed with Alizatin Red S and examined for skeletal defects. The authors observed no impact of tartaric acid on implantations, maternal, or foetal survival when administered at doses of up to 274 mg/kg body weight/day. The authors reported no significant differences between the number and nature of the abnormalities occurring the soft tissue or skeletal tissues of foetuses from the sham (negative control) group and the groups foetuses from pregnant mice treated with tartaric acid.
In the second study, adult Wistar-derived female albino rats were mated with young male rats in a protocol similar to that employed for the mice (FDRL, 1973). The females were individually housed and food and water was provided ad libitum. Administration of the test compounds began 0n the 6th day of the gestation period and continued through the 15th day of gestation. Groups of 19 to 24 female rats were administered either a vehicle (negative control), 250 mg/kg body weight/day of aspirin (positive control), or 1.81, 8.41, 39.1, or 181.0 mg tartaric acid/kg body weight/day. The negative control, positive control, and tartaric acid doses were provided in an administration volume that ranged between 1 and 6.4 mL/kg. During gestation the dams were examined daily and the food and water consumption was measured. Body weights were measured on Days 0, 6, 11, 15, and 20 of gestation. On the 20th day of gestation, the pups were delivered via caesarean section and the pups were examined. Parameters measured included were the same as those listed above for the mice. The authors observed no impact of tartaric acid on implantations, maternal, or foetal survival when administered at doses of up to 181 mg/kg body weight/day. The authors reported no significant differences between the number and nature of the abnormalities occurring in soft tissue or skeletal tissues of foetuses from the sham (negative control) group and foetuses from mice treated with tartaric acid.
The third study was conducted in the same manner as the first 2; however in this case the positive and negative controls and tartaric acid were administered to adult female golden hamsters. The administrations provided doses of 0 (vehicle control), 2.25, 10.45, 48.55, or 225.0 mg/kg body weight/day of tartaric acid or 250 mg/kg body weight/day of aspirin (positive control). The controls and test compounds were administered on Days 6 through 10 of gestation in a volume of 1 mL/kg body weight. The parameters examined were the same as those previously described for the studies conducted in mice and rats with the exception of body weights measured on Days 0, 6, 8, 10, and 14 of gestation and the caesarean sections initiated on Day 14 of gestation. The authors observed no impact of tartaric acid on implantations, maternal, or foetal survival when administered at doses of up to 225.0 mg/kg body weight/day. The authors reported no significant differences between the number and nature of the abnormalities occurring the soft tissue or skeletal tissues of foetuses from the sham (negative control) group and the groups foetuses from pregnant mice treated with tartaric acid.
The fourth and final study was conducted in the same manner as the first 3; however in this case the positive and negative controls and tartaric acid were administered to adult Dutch-belted female rabbits. In this case the virgin rabbits were artificially inseminated with semen from a proven donor buck. Prior to insemination, the rabbits were administered human chorionic gonadotropin to induce ovulation. The control and test compounds were administered by oral intubation on Days 6 through Day 29 of gestation. The administration provided doses of 0 (vehicle control), 2.15, 10.0, 46.4, or 215.0 mg/kg body weight/day of tartaric acid or 2.5 mg/kg body weight/day of 6-aminonicotinamide (positive control). The controls and test compounds were administered on Days 6 through 10 of gestation in a volume of 1 mL/kg body weight. The parameters examined were the same as those previously described for the studies conducted in mice and rats with the exception of body weights measured on Days 0, 6, 12, 18 and 29 of gestation and the caesarean sections initiated on Day 14 of gestation. The authors observed no impact of tartaric acid on implantations, maternal, or foetal survival when administered at doses of up to 215 mg/kg body weight/day. The authors reported no significant differences between the number and nature of the abnormalities occurring in the soft tissues or skeletal tissues of foetuses from the sham (negative control) group and the foetuses from mice treated with tartaric acid.
Iron
Physiological iron storage in adult human males and females is approximately 4.0 and 3.5 g, respectively (Bothwell et al., 1979). The majority of the physiological iron in adults is present as hemoglobin (60 to 70%) and myoglobin (10%), with the remainder (20 to 30%) located in storage pools in the liver and the reticuloendothelial system as ferritin and hemosiderin.
Approximately 1% of physiological iron is present in iron-containing enzymes, and <0.2% is present in the plasma transport pool, and is bound mostly to transferrin (SACN, 2010). Iron absorption occurs in the proximal small intestine, and absorption and transfer into the systemic circulation involves regulated iron uptake carrier proteins on the apical and basal surfaces of enterocytes (SACN, 2010). Non-heme iron is transported into the enterocyte by the divalent metal transporter 1 (DMT1), while the mechanism of heme iron absorption is unclear (SACN, 2010). In foods, non-heme iron is present as iron salts that dissociate upon ingestion. The absorption of iron is dependent on its dissolution and subsequent reduction to the ferrous form (Fe2+) (EFSA, 2004).
Iron absorbed from the gastrointestinal tract is transported in the systemic circulation as transferrin, which is composed of the carrier apotransferrin that binds to 1 or 2 atoms of ferric iron (Fe3+) to form holotransferrin (i.e. transferrin) (SACN, 2010). The liver is the main systemic depot of iron (SACN, 2010). Hepatocytes sequester iron in the soluble complex ferritin, or the insoluble derivative, hemosiderin. The highest concentrations of ferritin are found in the liver, spleen, and bone marrow, and serum concentrations of ferritin are typically between 15 and 300 μg/L (SACN, 2010).
The human body has no means of excreting excess iron; thus, iron absorption is tightly controlled. Loss of iron from the body occurs via desquamated skin cells, sweat, urine, gastrointestinal sections, hair, and menses (SACN, 2010).
JECFA has evaluated the safety of iron compounds, with the latest evaluations at the 68th meeting in 2007 and the 71st meeting in 2009 as part of the evaluations of sodium iron (III) ethylenediaminetetraacetate (EDTA) (JECFA, 2008a,b) and ferrous ammonium phosphate (JECFA, 2010a,b), respectively. These latest evaluations considered toxicological data on iron and iron compounds not previously evaluated. Thus, the toxicological monographs prepared for these compounds together with the previous monograph prepared at the 27th meeting in 1983 (JECFA, 1983) provide an up-to-date and comprehensive summary of the toxicological profile of iron. In these assessments, JECFA established and maintained a Provisional Maximum Tolerable Daily Intake (PMTDI) of 0.8 mg/kg body weight/day (i.e., 48 mg/day for a 60 kg individual) for all sources of iron with the exception of iron oxides used as coloring agents, or for iron supplementation during pregnancy, lactation, or for special clinical conditions.
The SCF and IOM have established RDAs of 8 to 18 mg iron/day for healthy adults, and the IOM and Health Canada have set recommended upper limit intakes of 45 mg iron/day (i.e., 0.75 mg iron/kg body weight for a 60 kg individual). In addition, 6 different iron (III) salts are GRAS for direct addition to foods as nutrient supplements, with no limitations on their use other than cGMP.
Ingestion of iron via consumption of the complexation products, under the intended conditions of use (i.e., ≤0.14 mg/person/day based on the consumption of 11.3 g salt/day), is considerably lower than intakes of iron from dietary sources, fortified foods, or supplements. In addition, iron (III) derived from the complexation products would be expected to be metabolized in the same way as other non-heme sources of iron. Considering the low level of exposure, it is expected that exposure to iron (III) via consumption of the complexation products will not pose any safety concerns.
Oxalate
Oxalic acid is naturally present in a wide variety of plant-based foods, such as fruits, vegetables, seeds, and cereal grains (Holmes and Kennedy, 2000; Massey et al. 2001; Siener et al., 2006; USDA, 2009). The presence of oxalic acid in plants is likely a result of oxidative degradation of ascorbic acid (Debolt et al., 2007). Oxalic acid also is a well-known oxidative product of ascorbic acid metabolism in humans (Burns, 1975), and is present in the human body following consumption of vitamin C or oxalic acid. Although oxalic acid is a normal dietary constituent, there exists concern that high dietary intake of oxalic acid may increase urinary oxalate excretion, thus increasing the risk of calcium oxalate precipitation in the kidneys (i.e., kidney stones) (Holmes et al., 2001). The potential exposure to oxalic acid from the complexation products under the intended conditions of use is considerably lower than that from other food ingredients such as citric acid. Consequently, there are no safety concerns associated with the presence of oxalic acid in the complexation products.
References
Bothwell TH, Charlton RW, Cook JD, Finch CA (1979).Iron Metabolism in Man. Oxford, UK: Blackwell Scientific Publications. Cited In: SACN, 2010.
Burns JJ (1975). Part I. Metabolism of ascorbic acid. introduction: overview of ascorbic acid metabolism. Annals of the New York Academy of Sciences 258: 5-6.
Debolt S, Melino V, Ford CM (2007).Ascorbate as a biosynthetic precursor in plants. Annals of Botany 99: 3-8.
EFSA (2004). Opinion of the Scientific Panel on Dietetic Products, Nutrition and Allergies on a request from the Commission related to the Tolerable Upper Intake Level of Iron (request no EFSA-Q-2003-018, adopted on 19 October 2004 by European Food Safety Authority). EFSA J 125:1-34. Available at:http://www.efsa.europa.eu/en/efsajournal/pub/125.htm.
FDRL (1973).Teratologic Evaluation of FDA 71-55 (Tartaric Acid). (PB 223 821). Submitted by Maspeth (NY): Food and Drug Research Labs. (FDRL), Inc. to Washington (DC): U.S. Food and Drug Administration (U.S. FDA).
Holmes RP, Kennedy M (2000). Estimation of the oxalate content of foods and daily oxalate intake. Kid Inter 57: 1662-1667
Holmes RP, Goodman HO, Assimos DG (2001).Contribution of dietary oxalate to urinary oxalate excretion. Kid Inter 59: 270-276.
JECFA (1983). Iron. In:Toxicological Evaluation of Certain Food Additives and Contaminants. 27th Meeting of the Joint FAO/WHO Expert Committee on Food Additives (JECFA), Apr. 11-20, 1983, Geneva, Switz. (WHO Food Additives Series, no 18). Geneva, Switz.: World Health Organization (WHO), pp. 203-219. Available at:http://www.inchem.org/documents/jecfa/jecmono/v18je18.htm.
JECFA (2008a). Sodium iron(III) ethylenediaminetetraacetic acid (sodium iron EDTA) (addendum). In:Safety Evaluation of Certain Food Additives and Contaminants. Sixty-eighth Meeting of the Joint FAO/WHO Expert Committee on Food Additives (JECFA), June 19-28, 2007, Geneva, Switz. (WHO Food Additive Series, no 59). Geneva, Switz.: World Health Organization (WHO), International Programme on Chemical Safety (IPCS), pp. 125-144, 452. Available at:http://whqlibdoc.who.int/publications/2008/9789241660594_eng.pdf.
JECFA (2008b). Evaluation of Certain Food Additives and Contaminants. Sixty-eighth Report of the Joint FAO/WHO Expert Committee on Food Additives (JECFA), June 19-28, 2007, Geneva, Switz. (WHO Technical Report Series, no 947). Geneva, Switz.: World Health Organization (WHO). Available at:http://whqlibdoc.who.int/publications/2007/9789241209472_eng.pdf.
JECFA (2010a). Ferrous ammonium phosphate. In:Safety Evaluation of Certain Food Additives. Seventy-first Meeting of the Joint FAO/WHO Expert Committee on Food Additives, June 16-24, 2009, Geneva, Switz. (WHO Food Additive Series, no 62). Geneva, Switz.: World Health Organization (WHO), pp. 57-117, 281-282. Available at:http://whqlibdoc.who.int/publications/2010/9789241660624_eng.pdf.
JECFA (2010b).Evaluation of Certain Food Additives. Seventy-first Meeting of the Joint FAO/WHO Expert Committee on Food Additives, June 16-24, 2009, Geneva, Switz. (WHO Technical Report Series no 956). Geneva, Switz.: World Health Organization (WHO). Available at:http://whqlibdoc.who.int/trs/WHO_TRS_956_eng.pdf.
Massey LK, Palmer RG, Horner HT (2001). Oxalate content of soybean seeds (Glycine max: Leguminosae), soyfoods, and other edible legumes. J Agr Food Chem 49: 4262-4266.
SACN (2010).Iron and Health. Published for the U.K. Department of Health, Scientific Advisory Committee on Nutrition (SACN) by London, Engl.: The Stationary Office (TSO). Available at:http://www.sacn.gov.uk/reports_position_statements/reports/sacn_iron_and_health_report.html.
Siener R, Hönow R, Voss S, Seidler A, Hesse A (2006).Oxalate content of cereals and cereal products. J Agr Food Chem 54: 3008-3011.
USDA (2009).Oxalic Acid Content of Selected Vegetables. U.S. Department of Agriculture (USDA), Agricultural Research Service (ARS), Human Nutrition Research Center Nutrient Data Laboratory, Beltsville, MD. Available from:http://www.ars.usda.gov/Services/docs.htm?docid=9444[Last Modified: 08/13/2009].
Justification for classification or non-classification
Reproduction and development were unaffected up to 2000 mg/kg body weight/day in an extended OECD422 screening study. Furthermore
data on the different components of the “Complexation products of sodium tartrate with iron trichloride” indicates there is no hazard.
Therefore classification and labeling is not warranted.Additional information
Information on Registered Substances comes from registration dossiers which have been assigned a registration number. The assignment of a registration number does however not guarantee that the information in the dossier is correct or that the dossier is compliant with Regulation (EC) No 1907/2006 (the REACH Regulation). This information has not been reviewed or verified by the Agency or any other authority. The content is subject to change without prior notice.
Reproduction or further distribution of this information may be subject to copyright protection. Use of the information without obtaining the permission from the owner(s) of the respective information might violate the rights of the owner.
