Registration Dossier
Registration Dossier
Data platform availability banner - registered substances factsheets
Please be aware that this old REACH registration data factsheet is no longer maintained; it remains frozen as of 19th May 2023.
The new ECHA CHEM database has been released by ECHA, and it now contains all REACH registration data. There are more details on the transition of ECHA's published data to ECHA CHEM here.
Diss Factsheets
Use of this information is subject to copyright laws and may require the permission of the owner of the information, as described in the ECHA Legal Notice.
EC number: 900-600-0 | CAS number: -
- Life Cycle description
- Uses advised against
- Endpoint summary
- Appearance / physical state / colour
- Melting point / freezing point
- Boiling point
- Density
- Particle size distribution (Granulometry)
- Vapour pressure
- Partition coefficient
- Water solubility
- Solubility in organic solvents / fat solubility
- Surface tension
- Flash point
- Auto flammability
- Flammability
- Explosiveness
- Oxidising properties
- Oxidation reduction potential
- Stability in organic solvents and identity of relevant degradation products
- Storage stability and reactivity towards container material
- Stability: thermal, sunlight, metals
- pH
- Dissociation constant
- Viscosity
- Additional physico-chemical information
- Additional physico-chemical properties of nanomaterials
- Nanomaterial agglomeration / aggregation
- Nanomaterial crystalline phase
- Nanomaterial crystallite and grain size
- Nanomaterial aspect ratio / shape
- Nanomaterial specific surface area
- Nanomaterial Zeta potential
- Nanomaterial surface chemistry
- Nanomaterial dustiness
- Nanomaterial porosity
- Nanomaterial pour density
- Nanomaterial photocatalytic activity
- Nanomaterial radical formation potential
- Nanomaterial catalytic activity
- Endpoint summary
- Stability
- Biodegradation
- Bioaccumulation
- Transport and distribution
- Environmental data
- Additional information on environmental fate and behaviour
- Ecotoxicological Summary
- Aquatic toxicity
- Endpoint summary
- Short-term toxicity to fish
- Long-term toxicity to fish
- Short-term toxicity to aquatic invertebrates
- Long-term toxicity to aquatic invertebrates
- Toxicity to aquatic algae and cyanobacteria
- Toxicity to aquatic plants other than algae
- Toxicity to microorganisms
- Endocrine disrupter testing in aquatic vertebrates – in vivo
- Toxicity to other aquatic organisms
- Sediment toxicity
- Terrestrial toxicity
- Biological effects monitoring
- Biotransformation and kinetics
- Additional ecotoxological information
- Toxicological Summary
- Toxicokinetics, metabolism and distribution
- Acute Toxicity
- Irritation / corrosion
- Sensitisation
- Repeated dose toxicity
- Genetic toxicity
- Carcinogenicity
- Toxicity to reproduction
- Specific investigations
- Exposure related observations in humans
- Toxic effects on livestock and pets
- Additional toxicological data
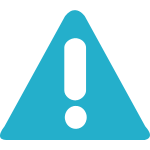
Endpoint summary
Administrative data
Link to relevant study record(s)
Description of key information
Absorption: readily absorbed by all routes (gastrointestinal, respiratory tracts, slowest through skin).
Distribution: all organs, fast and relative to the perfusion of organs with exception of fat (higher half-life)
Metabolism: mainly by cytochrome P-450 enzymes (CYP 2E1 and CYP3Ax), with the production of the trichloromethyl radical. Aerobically: trichloromethyl radical -> phosgene, carbondioxide; anaerobically: trichloromethyl radical -> chloroform, hexachloroethane or carbon monoxide; covalent binding of aerobic and anaerobic metabolites to lipids, proteins, and DNA possible.
Excretion: primarily in exhaled air and in the faeces, relatively minimal amounts in the urine
Short description of key information on absorption rate (studies not presented in this dossier but available in the CTC dossier):
Absorption rate: 53.6 ± 9.30 nmol/min/cm² skin for the mouse, no guideline followed; Tsuruta (1975) and 1.246 µmol/min/cm² for the rat, no guideline followed; Morgan (1991)
Key value for chemical safety assessment
- Bioaccumulation potential:
- no bioaccumulation potential
Additional information
The low but significant water solubility, the good fat solubility, and the low molecular weight (153.82 g/mol) of CTC result in a high membrane permeability and thereby a high absorption rate for the oral and the inhalation route and a reasonably fast absorption through skin is found for mammals. In spite of physico-chemical properties, the substance is not expected to have a bioaccumulation potential since half-lives in organs are low (between 4 and 12 hours). Most of following data come from the ATSDR Toxicological Profile of Carbon Tetrachloride (2005).
Absorption:
Carbon tetrachloride is readily absorbed by all routes (gastrointestinal, respiratory tracts, slowest through skin). It can be anticipated that carbon tetrachloride is well absorbed from the gastrointestinal tract in humans, since carbon tetrachloride is readily absorbed from the gastrointestinal tract of animals. In animals, the amount of gastro-intestinal absorption is estimated to be around 85 %. Oral absorption is influenced by the vehicle: when administerd in water or other aqueous vehicles, absorption of CTC is much more rapid and extensive than when administered in corn oil. Furthermore, peak arterial blood concentrations are reached within 15 minutes after bolus administration and then decline, whereas infusion causes a steady increase over the 2-hour period. Carbon tetrachloride is very rapidly absorbed from the lungs. As a small volatile haloalkane, carbon tetrachloride diffuses passively across cell membranes, leading to rapid absorption from the lungs and gastrointestinal tract into the circulatory system. Absorption across the lung is estimated to be about 60% in humans, from studies available in rodents and in monkey. Carbon tetrachloride is significantly absorbed through the skin of humans, though less readily than from the lung or gastrointestinal tract. The dermal absorption of carbon tetrachloride vapour can occur but is negligible. Absorption rate through skin is comprised between 53.6 ± 9.30 nmol/min/cm² for the mouse (Tsuruta 1975) and 1.246 µmol/min/cm² for the rat (Morgan 1991).
Distribution:
Carbon tetrachloride is distributed to all organs, the perfusion is fast for organs with exception of fat (accumulation and slow rise and fall of fat tissue concentrations).
Being somewhat lipophilic, absorbed carbon tetrachloride diffuses from the blood to the liver, kidney, brain, and other organs and accumulates in adipose tissue. Following absorption by the gastrointestinal tract, a first-pass effect is apparent through the liver, where carbon tetrachloride is biotransformed and adducts are formed from reactive metabolites binding to cell macromolecules.
In rats exposed to 1,000 ppm for 2 hours (receiving a dose of 179 mg/kg), the maximal concentration of carbon tetrachloride was reached within 30 minutes (the earliest time point) in the liver, kidney, lung, brain, heart, muscle, spleen, and gastrointestinal tract, and by 240 minutes in fat (Sanzgiri et al. 1997). The maximal concentration of carbon tetrachloride (μg/g tissue) achieved in the kidney was 1.25 times higher than the liver. When animals are dosed by oral route, the liver maximal concentration is increased; and CTC is distributed to same organs than by inhalation. In rats receiving a dose of 179 mg/kg by infusion over 2 hours, the maximal concentration of carbon tetrachloride was reached by 120 minutes in the liver, kidney, and heart, 150 minutes in the brain, muscle, and spleen, 180 minutes in lung, and by 360 minutes in fat (Sanzgiri et al. 1997).
Metabolism:
The metabolism of carbon tetrachloride in humans has not been investigated, but a great deal of information is available from studies in animals. Carbon tetrachloride is mainly metabolized by cytochrome P-450 enzymes (CYP 2E1 and CYP3A), with the production of the trichloromethyl radical, which has a subsequent aerobic metabolism: trichloromethyl radical -> phosgene, carbon dioxide; anaerobically: trichloromethyl radical -> chloroform, hexachloroethane or carbon monoxide; covalent binding of aerobic and anaerobic metabolites to lipids, proteins, and DNA possible. Haloalkylation and lipid peroxidation by metabolites accounted for the mode of action of CTC toxicity in liver and kidney.
Excretion:
Carbon tetrachloride is primarily exhaled in air and excreted in faeces, and with relatively minimal amounts in urine. In humans and animals exposed to carbon tetrachloride by any route, the unmetabolized parent compound is excreted in exhaled air. Additionally, animal studies show that volatile metabolites are released in exhaled air, whereas non-volatile metabolites are excreted in faeces and to a lesser degree, in urine. After inhalation exposure, the clearance is relatively comparable from liver, kidney, lung, brain, heart, muscle and spleen, and significantly lower for fat. Same observation has been made after oral exposure, with differences in clearance of liver, depending whether on a bolus absorption or a gastric infusion.
Discussion on bioaccumulation potential result:
In addition to the study of Sanzgiri (1997) which is regarded as key study, many studies on the toxicokinetics of CTC have been carried out in the past 30 years. Following gastric infusion of 179 mg/kg of CCl4 in rats, the half-life in fat was about 12 hours, whereas the half-lives in liver, kidney, lung, brain, heart, muscle and spleen were around 4 hours. Other findings are summarized in the citations from the ATSDR dossier 2005. CTC has a low but significant water solubility and a good fat solubility, has a low molecular weight (153,82 g/mol), it has a high membrane permeability and thereby a high absorption rate for the oral and the inhalation route and a reasonably fast absorption trough skin is found for mammals. The log Pow (2.83) is compatible with an accumulation in fat. Nevertheless the bioaccumulation potential is expected to be extremely low regarding the half-lives of CTC in many organs. As shown in Sanzgiri (1997), absorption of CTC is fast through either the gastro intestinal tract or the lung (peak levels 30 min after start of exposure) except for the fat where maximal levels were reached later (after 2h in this test system). The same is reported for dermal absorption (see 7.1.2). CTC is distributed to all major organs, with highest concentrations in the fat, liver, bone marrow, adrenals, blood, brain, spinal cord, and kidney. Inhalation studies in monkeys, rats, and hamsters and mice reveal that the highest CTC concentrations occur in fat, and in organs or tissues with high fat content such as bone marrow, liver, brain, and kidney. The tissue concentrations reached are proportional to the diffusion rate of the organs and their fat content (highest concentrations in the fat, liver, bone marrow, adrenals, blood, brain, spinal cord, and kidney). Comparing inhalation and continuous gastric infusion a first pass effect becomes obvious, reducing CTC blood levels by metabolism. Once absorbed, CTC is metabolized by cytochrome P-450 enzymes with the production of the trichloromethyl radical: aerobically, metabolism of the trichloromethyl radical can eventually form phosgene; anaerobically, the radical can undergo reactions to form chloroform, hexachloroethane, as well as bind directly to lipids, proteins, and DNA. As found in other studies summarized in the ATSDR dossier, CTC is metabolized mainly by CYP2E1 and to a lower extent by other Cytochrome P450 enzymes. An electron is transferred to CTC to form chloride and a carbon trichloride radical. This reactive intermediate can react with oxygen to form trichloromethylperoxy radical and subsequently phosgene, can dimerise to hexachloroethane or can react with a proton and electron to form chloroform. Reductive dechlorination would lead to carbon monoxide. The trichloromethyl radical, the trichloromethylperoxy radical, phosgene and subsequent metabolites of chloroform can covalently modify biomolecules. Especially haloalkylation and lipid peroxidation are attributed to the toxicity of CTC seen in the metabolic active tissues of liver and kidneys. According to Draper (2009) the conversion rate of CTC in the liver is low compared to exhalation in the rat. Excretion occurs primarily in exhaled air and in the faeces, while relatively minimal amounts are excreted in the urine. Depending on species and study design values of 34 -75% excretion via exhalation and 20 -62% excretion via faeces and only minor excretion via the urine have been determined (ATSDR 2005).
Discussion on absorption rate:
No reliable study is available that is compliant to OECD TG 427 or 428. The stated value for the mouse and the rat are based on different methods and are different by a factor of about 40. The value derived from Morgan (1991) might be overestimated due to diffusion loss from the reservoir and can therefore be regarded as a worst-case scenario. The curves of blood concentration levels against time have similar shapes for guinea pigs and rats with a fast rise, a maximum after 30 min and then a slow decrease towards a steady state value. The decrease after the initial maximum at 30 min might be explicable by a reaction of the treated skin reducing the blood flow through this region and hydropic swelling. The area of exposure is similar in the rat and the guinea pig study (3.1 cm²) so if different body weights for the two species are taken into account the CTC blood levels are comparable in the two studies (Jakobson 1982, guinea pig: 1 µg/mL at 30 min, 0.26 0181 µg/mL after 6 h; Morgan 1991, F344 rat: 5 µg/mL at 30 min, 3.5 µg/mL after 6 h). The higher general permeability of rat skin might well account for the remaining difference. Though not following any guideline together these studies show that CTC is readily absorbed through the rat skin with a rate of 1.246 µmol/min/cm².
Information on Registered Substances comes from registration dossiers which have been assigned a registration number. The assignment of a registration number does however not guarantee that the information in the dossier is correct or that the dossier is compliant with Regulation (EC) No 1907/2006 (the REACH Regulation). This information has not been reviewed or verified by the Agency or any other authority. The content is subject to change without prior notice.
Reproduction or further distribution of this information may be subject to copyright protection. Use of the information without obtaining the permission from the owner(s) of the respective information might violate the rights of the owner.
