Registration Dossier
Registration Dossier
Data platform availability banner - registered substances factsheets
Please be aware that this old REACH registration data factsheet is no longer maintained; it remains frozen as of 19th May 2023.
The new ECHA CHEM database has been released by ECHA, and it now contains all REACH registration data. There are more details on the transition of ECHA's published data to ECHA CHEM here.
Diss Factsheets
Use of this information is subject to copyright laws and may require the permission of the owner of the information, as described in the ECHA Legal Notice.
EC number: 207-306-5 | CAS number: 460-19-5
- Life Cycle description
- Uses advised against
- Endpoint summary
- Appearance / physical state / colour
- Melting point / freezing point
- Boiling point
- Density
- Particle size distribution (Granulometry)
- Vapour pressure
- Partition coefficient
- Water solubility
- Solubility in organic solvents / fat solubility
- Surface tension
- Flash point
- Auto flammability
- Flammability
- Explosiveness
- Oxidising properties
- Oxidation reduction potential
- Stability in organic solvents and identity of relevant degradation products
- Storage stability and reactivity towards container material
- Stability: thermal, sunlight, metals
- pH
- Dissociation constant
- Viscosity
- Additional physico-chemical information
- Additional physico-chemical properties of nanomaterials
- Nanomaterial agglomeration / aggregation
- Nanomaterial crystalline phase
- Nanomaterial crystallite and grain size
- Nanomaterial aspect ratio / shape
- Nanomaterial specific surface area
- Nanomaterial Zeta potential
- Nanomaterial surface chemistry
- Nanomaterial dustiness
- Nanomaterial porosity
- Nanomaterial pour density
- Nanomaterial photocatalytic activity
- Nanomaterial radical formation potential
- Nanomaterial catalytic activity
- Endpoint summary
- Stability
- Biodegradation
- Bioaccumulation
- Transport and distribution
- Environmental data
- Additional information on environmental fate and behaviour
- Ecotoxicological Summary
- Aquatic toxicity
- Endpoint summary
- Short-term toxicity to fish
- Long-term toxicity to fish
- Short-term toxicity to aquatic invertebrates
- Long-term toxicity to aquatic invertebrates
- Toxicity to aquatic algae and cyanobacteria
- Toxicity to aquatic plants other than algae
- Toxicity to microorganisms
- Endocrine disrupter testing in aquatic vertebrates – in vivo
- Toxicity to other aquatic organisms
- Sediment toxicity
- Terrestrial toxicity
- Biological effects monitoring
- Biotransformation and kinetics
- Additional ecotoxological information
- Toxicological Summary
- Toxicokinetics, metabolism and distribution
- Acute Toxicity
- Irritation / corrosion
- Sensitisation
- Repeated dose toxicity
- Genetic toxicity
- Carcinogenicity
- Toxicity to reproduction
- Specific investigations
- Exposure related observations in humans
- Toxic effects on livestock and pets
- Additional toxicological data
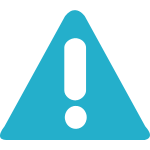
Endpoint summary
Administrative data
Link to relevant study record(s)
- Endpoint:
- dermal absorption in vivo
- Type of information:
- experimental study
- Adequacy of study:
- key study
- Reliability:
- 2 (reliable with restrictions)
- Rationale for reliability incl. deficiencies:
- study well documented, meets generally accepted scientific principles, acceptable for assessment
- GLP compliance:
- no
- Specific details on test material used for the study:
- ethanedinitrile 99.5%
- Radiolabelling:
- no
- Species:
- rabbit
- Strain:
- not specified
- Sex:
- male
- Type of coverage:
- open
- Vehicle:
- other: air
- Duration of exposure:
- 8h
- Doses:
- 10,000 ppm
- No. of animals per group:
- 4
- Control animals:
- yes
- Details on study design:
- Four male albino rabbits whose weights averaged 4.5 pounds were closely clipped of their fur, restrained in a wooden stock, and placed in the chamber. An especially designed door, through which the rabbits heads extended into glass jarsmaintained under positive air pressure, prevented the rabbits from breathing cyanogen while their bodies were being exposed and also made observation of the heads of the rabbits possible. The bodies of the rabbits were exposed to ethanedinitrile concentration of 10,000 ppm for a period of 8 hours.
- Signs and symptoms of toxicity:
- no effects
- Dermal irritation:
- no effects
- Conclusions:
- No dermal absorption was observed after exposure of rabbit to 10,000 ppm of ethanedinitrile.
- Executive summary:
Materials and methods
Four male albino rabbits whose weights averaged 4.5 pounds were closely clipped of their fur, restrained in a wooden stock, and placed in the chamber. An especially designed door, through which the rabbits heads extended into glass jarsmaintained under positive air pressure, prevented the rabbits from breathing cyanogen while their bodies were being exposed and also made observation of the heads of the rabbits possible. The bodies of the rabbits were exposed to ethanedinitrile concentration of 10,000 ppm for a period of 8 hours.
Conclusion
No dermal absorption was observed after exposure of rabbit to 10,000 ppm of ethanedinitrile.
- Endpoint:
- basic toxicokinetics
- Type of information:
- read-across based on grouping of substances (category approach)
- Adequacy of study:
- supporting study
- Reliability:
- 2 (reliable with restrictions)
- Rationale for reliability incl. deficiencies:
- other: see 'Remark'
- Remarks:
- Experimental data was reviewed by the ECETOC Task Force, author of the JACC Report No. 53, “Cyanides of Hydrogen, Sodium and Potassium, and Acetone Cyanohydrin (CAS No. 74-90-8, 143-33-9, 151-50-8 and 75-86-5)”, 2007. The report is a weight of evidence approach to an extensive body of literature, much of which was undertaken prior to development of guidelines. The report was peer reviewed by the scientific non-governmental organization (NGO), which judged the data to be reliable with restrictions.
- Justification for type of information:
- Ethanedinitrile, hydrogen cyanide (HCN), potassium cyanide and sodium cyanide can be considered as a chemical category, along with and acetone cyanohydrin (ACH, also known as 2-hydroxy-2-methylpropanenitrile), based on structural similarity, common breakdown/metabolic products in physical and biological systems, and similar physico-chemical properties. Particular attention is paid to the dissociation constant of HCN. Ethanedinitrile breaks down in aqueous solution into cyanide ion (CN-) and cyanate ion (OCN-) (Cotton and Wilkinson 1980). Ethanedinitrile due to its low log Kow (0.07) and relatively high solubility in water (2.34 g/L) needs to get dissolved in aqueous solutions in lungs to enter the body. The rate of hydrolysis of ethanedinitrile is very fast (Ajwa 2015). Also, in the vast majority of environmental and physiologic conditions, the cyanide salts will dissolve in water to form hydrogen cyanide. The physico-chemical hazards and toxicity therefore result from the activity of HCN. An ECETOC Task Force, in the 2007 ECETOC Joint Assessment of Commodity Chemicals (JACC) Report No. 53, “Cyanides of Hydrogen, Sodium and Potassium, and Acetone Cyanohydrin (CAS No. 74-90-8, 143-33-9, 151-50-8 and 75-86-5)” supports the development of the chemical category inclusive hydrogen cyanide, sodium and potassium cyanides. Hydrogen cyanide (Index No.006-006-00-X) and salts of hydrogen cyanides (Index No.006-007-00-5) are both listed in Annex VI, Table 3.1 of Regulation (EC) No. 1272/2008, entry 006-007-00-5, and are restricted in comparable ways taking into account physical characteristics. Thus, the assignment of ethanedinitrile to a chemical category does not result in a less protective regulatory status.
- Qualifier:
- no guideline followed
- Principles of method if other than guideline:
- Comprehensive literature review
- GLP compliance:
- no
- Conclusions:
- Interpretation of results (migrated information): other: The mechanism of action of cyanide is via binding to and inactivating cytochrome oxidase, causing cellular anoxia.
Hydrogen cyanide through the CN- ion binds to and inactives cytochrome oxidase, unleashing a cascade of metabolic effects which explain the clinical pathology of acute cyanide poisoning or chronic adaptive effects. Hydrogen cyanide (Index No.006-006-00-X) and salts of hydrogen cyanides (Index No.006-007-00-5) are both listed in Annex VI, Table 3.1 of Regulation (EC) No. 1272/2008, entry 006-007-00-5, and are restricted in comparable ways taking into account physical characteristics. Thus, the assignment of ethanedinitrile to a chemical category does not result in a less protective regulatory status.
Referenceopen allclose all
Cyanide poisoning is caused by complex formation with the iron in cytochrome oxidase which is present in tissues at cellular level. The complex formation inhibits oxygen from receiving electrons from the cytochrome oxidase and a so-called intracellular or cytotoxic anoxia occurs, i.e. oxygen is present but cannot be utilised by the cell. Because neurons and cardiac myocytes are highly dependent on aerobic metabolism they are extremely sensitive to the deprivation of oxygen. Anoxia leads to an inhibition of oxidative glucose metabolism in favour of anaerobic pathways, such as the pentosephosphate shunt and non-enzymatic pyruvate formation, and results in an increased formation of NADPH and lactate. At the cellular level, ATP depletion leads to an inhibition of Ca2+ and Ca2+/Mg2+ dependent K-Na-ATPases that regulate cytosolic levels of calcium ions. Increase of cytosolic calcium triggers a number of other processes that have been observed following cyanide exposure.
1. Formation of reactive oxygen species (ROS) and NO is mediated by an activation of phosphokinase C (PKC). PKC activates phospholipase A2 (PLA2) and NO synthetase. The latter leads to an increased NO formation.
2. Differentiated neuronal cells have been demonstrated to be more sensitive to ROS-induced apoptotic cell death following cyanide exposure than undifferentiated neuronal cells.
3. Cyanide-mediated elevated intracellular calcium levels lead to an activation of brain xanthine oxidase, thereby inducing an inhibition of antioxidative enzymes such as catalase, GSH dependent peroxidase and GSH reductase, and consequently depletion of reduced glutathione (GSH). This implies that the cells’ ability to neutralise increased levels of ROS is also impaired adding to the effect of lipid peroxidation as described under 1.
4. Elevated intracellular calcium levels also cause the increased release of excitatory neurotransmitters like dopamine and noradrenaline from granular stores within neuronal cells in brain and periphery. Adrenal catecholamine secretion may also be enhanced as a secondary effect. This contributes to an increased energy demand of the organism that aggravates the consequences of cyanide-induced ATP reduction and leads to a spread of the cytotoxic effect to other energy demanding organs, such as the heart which are less sensitive to the primary effect of cyanides.
The secondary mechanism of action relevant to repeated exposure to cyanide is formation of the primary metabolite thiocyanate. The main target for thiocyanate is the thyroid gland. Thiocyanate is a competitive inhibitor of the iodine uptake by the thyroid, causing depletion of iodine in the thyroid depending on the ratio of thiocyanate and iodine levels in blood. Reduced intra-thyroidal iodine levels result in reduced thyroid hormone synthesis, excretion and blood levels, in particular tri-iodothyronin (T3) and serum thyroxin (T4). The lower T3 and T4 levels, in turn, up-regulate the hypothalamus, increasing secretion of thyrotropin releasing hormone (TRH). This leads to an increased secretion of thyroid stimulating hormone (TSH) by the pituitary and an increase in hormone producing cells of the thyroid that may result in hyperthyroidism (goitre) formation.
Description of key information
Studies on Absorption, Distribution, Metabolism and Excretion in Mammals
Ethanedinitrile has same mode of action as other compounds with cyanide like toxicity as hydrogen cyanide (HCN) or acetonitrile (Dzombak et al. 2006; Hartung 1994; ATSDR 2006). They prevent use of oxygen in mitochondria by blocking cytochrome c oxidase ultimately leading to asphyxiation on cellular level (Dzombak et al. 2006; Pelclová 2014). CN-ion is the part of molecule responsible for the toxic action and it is therefore possible to compare the relative toxicity of these compounds based on ratio of their molecular weight to CN-ion (Dzombak et al. 2006). Consequently, it is possible to use and interchange toxicological data of chemicals within this group (Dzombak et al. 2006) commonly referred in a regulatory toxicology as a read across approach. For instance, toxicological data of ethanedinitrile were successfully used instead of HCN data in biocidal registration of this active compound (Commision Directive 2012/42/EU). Nevertheless, it is appropriate to consider different molecular weights of these substances and also other physical and chemical properties, e.g. some routes of exposure are not possible for some compounds, since e.g. EDN is a gas and acetonitrile and HCN is liquid. It is important to keep in mind that although the mode of action of these compounds i.e. toxicodynamics is same (thus once they are in blood stream they will act similarly from toxicological point of view), they are very different compounds due to its very different physical and chemical characteristics.
The only exposure route for ethanedinitrile is inhalation. The oral route for cyanides is described due to the fact that ethanedinitrile breaks down in aqueous solutions into the same compounds as are involved in metabolism and breakdown of cyanides (Figure 8.8.-1). Metabolism of HCN is well described in scientific literature using mainly data from human case studies with several supporting studies on animals. It is not necessary to conduct new metabolisms studyin vivo when relevant human data are present and indicative in vitro study for such research would be also redundant. Therefore, part of distribution, metabolism and excretion of oral exposure to cyanides applies also for inhalation exposure to ethanedinitrile. |
Absorption, distribution, metabolism and excretion by oral exposure
Absorption:
Ethanedinitrile Ethanedinitrile can be taken only by inhalation route due to its physical characteristic – the boiling point of the ethanedinitrile is -21 °C. Cyanides All cyanides ingested will be present, at the physiological pH of the stomach, as HCN.The HCN will be absorbed in the blood via the stomach wall and in the intestines. Before the blood enters the systemic circulation, it has to pass the liver with a relatively high content of the enzyme rhodanese. A considerable part of cyanide is detoxified in the liver (JACC 2007). This is apparent from comparison of the oral LD50value for rabbits (0.092 mmol/kg bw) with those obtained by intravenous (i.v.) injection or inhalation for 5 minutes (0.022 or 0.019 mmol/kg bw, respectively) (Ballantyne 1987a), indicating a first-pass effect via ingestion.
Limited data are available on oral absorption of cyanide in humans. In some cases of human ingestion, victims have become unconscious within seconds and died within minutes of exposure. The rate of absorption of cyanide from the gastrointestinal tract depends on the chemical form of the cyanide and the presence of food in the tract. Food in the stomach significantly delays the absorption of cyanide, and experimental results have demonstrated that dogs and cattle can be protected from the lethal effects of cyanide by the presence of carbohydrates in the stomach (Liebowitz and Schwartz 1948).
Gettler and Baine (1938) reported data indicating that, at doses above historical lethal doses, absorption decreases with increasing dose levels. Absorption was estimated at 19.5, 18.1, and 15.7 % in people estimated to have ingested 297 mg, 557 mg, and 1,450 mg HCN in suicide attempts. The low absorption at the highest dose may have been due to death occurring before absorption was complete. The assumption that absorption is lower at higher lethal doses is supported by a case report where absorption was approximately 82% in an individual, estimated as having ingested 30 mg HCN, who died more than 3 hours after exposure (Table 8.8.-1).
Animal data also indicate extensive absorption. In male Sprague-Dawley rats treated by gavage with 1 mg/kg KCN, a peak blood level (Cmax) of 6.2 nmol/mL CN–(160 μg/L) was observed 2 minutes after treatment (Tmax),and a blood elimination half-life (t1/2) of 14.1 min was calculated from the pharmacokinetic data obtained(Leuschner et al. 1991,Table 8.8.-1).
Oral absorption was essentially comparable (16.6 and 15.7%) in dogs that ingested 100 and 50 mg HCN (as cyanides), respectively. However, absorption increased to 72 % in a dog that ingested 20 mg HCN (1.5 mg/kg) (as cyanides)Gettler and Baine (1938).
Distribution:
Ethanedinitrile Ethanedinitrile can be taken only by inhalation route due to its physical characteristic – the boiling point of the ethanedinitrile is -21 °C. Cyanides Limited data on distribution of cyanide in humans, following oral exposure, are available. Cyanide distributes rapidly and uniformly throughout the body following absorption. Immediately following oral cyanide exposure, the stomach contents appear to contain the highest concentration of cyanide. Other tissues containing cyanide included the liver, brain, spleen, blood, kidneys, and lungs (Ansell and Lewis 1970; Gettler and Baine 1938). A number of illustrative levels of cyanide in organs and blood after oral intake in humans (Ansell and Lewis 1970; ATSDR 1997) and rabbits (Ballantyne 1983a) have been reported. For a given exposure route, whole blood and serum cyanide levels are quite similar for different species (Ballantyne 1983a). Okoh and Pitt (1982) investigated the tissue distribution of cyanide in rats fed KCN in the diet at 77 μmol/day (approximately 5.5 mg/kg per day CN-) for 3 weeks and then injected intraperitoneally (i.p.) with radiolabelled NaCN. Radioactivity was widely distributed, with the highest concentrations in the gastrointestinal tract, blood, kidneys, lungs, spleen, and liver. Radioactivity appeared in the stomach as early as 10 minutes after injection, with 18% of the injected dose found in the stomach contents within 60 minutes of dosing. More than 80% of the radioactivity in the stomach was present as thiocyanate (SCN-), with small portions present as cyanide and radiolabelled carbon dioxide.
In one study, distribution of CN-was investigated by per-os treatment of rats. Rats received cyanide radical of 7 mg or 21 mg/kg body weight as NaCN solution that was injected into stomach. Rats died after 10.3 minutes for 7 mg group and after 3.3 minutes for 21 mg group. When both groups were combined, the concentration decreased in order: liver, lungs, spleen, blood (only slightly lower) and brain. (Yamamoto et al. 1982,Table 8.8.-1).
Metabolism:
Ethanedinitrile Ethanedinitrile can be taken only by inhalation route due to its physical characteristic – the boiling point of the ethanedinitrile is -21 °C.
Cyanides Although cyanide can interact with substances such as methaemoglobin in the bloodstream, the majority of cyanide metabolism occurs within the tissues. Cyanide is metabolized in mammalian systems by one major route (Figure 8.8.-1) and several minor routes. The major route of metabolism for hydrogen cyanide and cyanides is detoxification in the liver by the mitochondrial enzyme rhodanese, which catalyses the transfer of the sulfane sulfur of thiosulfate to the cyanide ion to form thiocyanate (Williams 1959; Ansell and Lewis 1970). About 80 % of cyanide is detoxified by this route. The rate-limiting step is the amount of thiosulfate. While rhodanese is present in the mitochondria of all tissues, the species and tissue distributions of rhodanese are highly variable. In general, the highest concentrations of rhodanese are found in the liver, kidney, brain, and muscle, but the supply of thiosulfate is limited (Aminlari et al. 1994). Rhodanese is present in rat nasal mucosal tissues, particularly in the olfactory region, at a 7-fold higher concentration (on a per milligram of mitochondrial protein basis) than in the liver (Dahl 1989). Dogs have a lower overall activity of rhodanase than monkeys, rats, and rabbits (ATSDR 2006). |
Excretion: Half-time values of the principal metabolite thiocyanate in humans have been reported as 4 h (Blaschle and Melmon 1980), 2 days (Bödigheimer et al. 1979), and 2.7 days (Schulz et al. 1979). In patients with renal insufficiency, a mean half-time of 9 days was reported (Bödigheimer et al. 1979). Urinary excretion of thiocyanate was monitored in a man after ingestion of about 3–5 g potassium cyanide (15–25 mg cyanide/kg bw) (Liebowitz and Schwartz 1948; ATSDR 1997). The results indicated that the patient excreted 237 mg of thiocyanate over a 72-h period. This quantity was substantially more than the normal average amount of thiocyanate in urine, which varies from 0.85 to 14 mg/24 h (ATSDR 1997,Table 8.8.-1).
While absorbed cyanide is principally excreted as thiocyanate in the urine, traces of free HCN may also be excreted unchanged in the lungs, saliva, sweat, or urine (Hartung 1982), as carbon dioxide in expired air, or as β-thiocyanoalanine (minor path, ATCA – 2-Aminothiazoline-4-carboxylic acid) in saliva and sweat (Friedberg and Schwartzkopf 1969; Hartung 1982; JECFA 1993).
There is also important role of thiocyanate in cystic fibrosis and inflammatory diseases. It is probable that insufficient levels of antioxidant thiocyanate (caused by cystic fibrosis) provide inadequate protection from hypochlorite (OCl(-)) and hydrogen peroxide (H2O2), thus worsening inflammatory diseases, and predisposing humans to several diseases including atherosclerosis, neurodegeneration, and certain cancers (Xu et al. 2009).
The limiting factor in cyanide metabolism is the low concentration of the sulfur-containing substrates in the body – primarily thiosulfate, but also cystine and cysteine. The rate of spontaneous detoxification of cyanide in humans is about 1 μg/kg bw per minute (Schulz 1984), which is considerably slower than in small rodents (Schubert and Brill 1968) or dogs (Lawrence 1947). After administration of an intravenous dose of 3‑4 mg potassium cyanide to beagle dogs, blood levels decreased in a manner consistent with first-order elimination kinetics for the first 80 min (Bright and Marrs 1988). The half-time for this phase was about 24 min, corresponding to an elimination rate constant of 0.03/min. After 80 min, the blood cyanide concentrations fell at a slower rate, with a half‑time of 5.5 h. |
In rats, after a single oral dose, the blood elimination half-time of cyanide was 14.1 min, corresponding to a rate constant of 0.05/min (Leuschner et al. 1991). Rats treated orally with 2 mg cyanide/kg bw excreted 47 % of the dose in the urine within 24 h (Farooqui and Ahmed 1982,Table 8.8.-1). A [14C] cyanide intake study with rats (exposed to a regular intake of cyanide in the diet for 3 weeks) indicated the existence of a gastrointestinal circulation of thiocyanate, in which a substantial amount of thiocyanate, which was excreted into the stomach contents of the rat, was reabsorbed by the intestine into the body fluid, to be partly excreted in the urine and partly resecreted into the gastric contents (Okoh and Pitt 1982). The relative proportion of cyanide to thiocyanate in body fluids is about 1:1000 (Pettigrew and Fell 1973). The half-time for hydrogen cyanide elimination is about 1 h (Ansell and Lewis 1970, IPCS 1992).
Absorption, distribution, metabolism and excretion by other routes
Absorption:
Ethanedinitrile
Inhalation Absorption of ethanedinitrile occurs through lungs.
Dermal Ethanedinitrile has no absorption through skin, exposure of 10,000 ppm (~ 21 g/m3) for eight hours, did not resulted any clinical observations or macroscopic effects on rabbit (McNerney and Schrenk 1960).
Cyanides
Inhalation Only limited data are available on absorption of inhaled cyanide by humans. Landahl and Herrmann (1950) measured the pulmonary retention of HCN in 10 volunteers exposed to concentrations of 0.0005–0.02 mg/L (0.5–20 mg/m3) for up to 3 minutes. All subjects breathed through their mouths. The percent retained in the lung (and, presumably, the percent absorbed) was approximately 60% and ranged from 58 to 77% among people who were breathing normally. Rapid, shallow breathing appeared to decrease absorption.
Quantitative data on the absorption of hydrogen cyanide by inhalation were reported in dogs (Gettler and Baine 1938). During exposure to hydrogen cyanide, one dog reportedly absorbed 16.0 mg (1.55 mg/kg); the other dog absorbed 10.1 mg (1.11 mg/kg). These doses were fatal to the dogs in 12 and 8 minutes, respectively; seeTable 8.8.-2.
Dermal Liquidcyanide compoundsare easily absorbed through intact skin upon direct contact due to their lipid solubility and rapid epidermal penetration. Skin absorption of vapours of hydrogen cyanide is also possible when the air concentrations are high. The amount and rate of absorption of cyanides from aqueous solutions or atmospheric hydrogen cyanide depend upon the presence of moisture in the skin, concentration and pH of the solution, the surface area of contact, and the duration of contact (Dugard 1987).In vitrostudies with human skin have shown that penetration of sodium cyanide in aqueous solution through skin decreases with increasing pH (increasing dissociation), reflecting the more rapid absorption of the undissociated hydrogen cyanide. The permeability constant measured for the cyanide ion in aqueous solution was 3.5 × 10–4cm/h, and that calculated for hydrogen cyanide was 1 × 10–4cm/h (Dugard 1987).
Dermal absorption of HCN gas has also been observed in humans. Drinker (1932) reported that three workers working in an atmosphere containing 2% HCN (20,000 ppm; 22,100 mg/m3) for 8–10 minutes became dizzy and weak and were on the verge of unconsciousness, despite wearing gas masks providing excellent respiratory protection. The observed effects were attributed to dermal absorption of the gas. Potter (1950) reported on a worker wearing respiratory protection and protective clothing whose hands were accidentally exposed to liquid HCN. Within 5 minutes, the worker became dizzy, had difficulty breathing, and fell unconscious.
Information regarding dermal absorption of cyanide in animals was provided in studies of guinea pigs and dogs. In a dermal toxicity study, shaved area of abdominal skin of 8 guinea pigs has been exposed to saturated vapours of HCN. Clinical symptoms of toxicity and autopsy results were the same in all animals and were observed after 6–7 min – rapid respiration, general twitching of muscles, convulsions. All exposed animals died at 7–8 min (Walton and Whiterspoon 1925;Table 8.8.-2). Similar study of dermal toxicity was performed with whole body (except head) exposure of 11 dogs to HCN vapours in concentrations of 5.5 to 16.9 mg/L air. Dogs tolerated a concentration of 5.5 mg/l for up to 180 minutes without any ill effects. Clinical signs of toxicity (muscle twitching) appeared in animals exposed to HCN concentrations 10.9 mg/L and higher. At concentrations 11.6 mg/L and higher (concentration x time product values of 11 g.h.m-3and higher) 6 of 7 animals died (one of them was euthanized) (Walton and Whiterspoon 1925;Table 8.8.-2).
Distribution:
Ethanedinitrile Inhalation Ethanedinitrile breaks down in aqueous solution into cyanide ion (CN-) and OCN-ions (Yngard et al. 2008, Cotton and Wilkinson 1980). Ethanedinitrile due to its low log Kow (0.07) and relatively high solubility in water (2.34 g/L) is expected to get dissolved in aqueous solutions in lungs to enter the body. The rate of hydrolysis of ethanedinitrile is very fast (Ajwa 2015).
Therefore, inhalation of ethanedinitrile leads to exposure to HCN (ATSDR 2006). The products of hydrolyses are hydrogen cyanide and cyanic acid (HOCN) (Cotton and Wilkinson 1980). Both of these compounds are already included in the ADME process of hydrogen cyanide in the body; see Figure 8.8.-1 (ATSDR 2006). Thus, the distribution described in this section (5.1.2) for cyanides and in section 5.1.1 for cyanides administred by oral route is also partialy relevant for ethanedinitrile.
Cyanides (HCN)
Inhalation Cyanide distributes rapidly and uniformly throughout the body following absorption. Once cyanide is absorbed, it is distributed by the blood throughout the body. Tissue levels of hydrogen cyanide were 0.75, 0.42, 0.41, 0.33, and 0.32 mg/100 g of tissue in the lung, heart, blood, kidney, and brain, respectively, in a man who died following inhalation exposure to hydrogen cyanide gas (Gettler and Baine 1938;Table 8.8.-2.
In another case, tissue cyanide levels from a man who died from inhalation of hydrogen cyanide were reported as 0.5 mg per 100 mL of blood and 0.11, 0.07, and 0.03 mg/100 g in the kidney, brain, and liver, respectively (Gettler and Baine 1938;Table 8.8.-2.
Following chronic occupational exposure to 0.19–0.75 ppm hydrogen cyanide, 56.0 µg CN-/100 mL (23.2 µg/100 mL when outlying value - 220 µg/100 mL - was excluded) was found in blood of smokers and 18.3 μg CN-/100 mL in non-smokers (Chandra et al. 1980). The cyanide levels in control groups were 4.8 μg/100mL for smokers and 3.2 μg/100mL for non-smokers;Table 8.8.-2.
In two dogs exposed to unspecified fatal concentrations of hydrogen cyanide, the highest cyanide levels were found in the lungs, blood, and heart (Gettler and Baine 1938).
Rats exposed to hydrogen cyanide gas at 356 ppm or 1,180 ppm died within 10 and 5 minutes, respectively (Yamamoto et al. 1982). Samples, taken immediately after respiration stopped, showed that the pattern of tissue distribution of cyanide did not vary with the concentration used. In averaging data for both dose groups, tissue concentrations, reported as μg/g wet weight (ww), were 4.4 in the lungs, 3.0 in the blood, 2.15 in the liver, 1.4 in the brain, and 0.68 in the spleen. Thus, the highest cyanide concentrations were observed in the lung;seeTable 8.8.-2.
Rabbits exposed to hydrogen cyanide at 2,714 ppm for 5 minutes had cyanide levels of 170/100 μg/mL in blood and 48 μg/100 mL in plasma, and tissue levels (in units of μg/100 g) of 0 in the liver, 6 in the kidney, 50 in the brain, 62 in the heart, 54 in the lung, and 6 in the spleen (Ballantyne 1983a;Table 8.8.-2).
Cyanate Most of the cyanate is broken to CO2(72%) 24 hours after injection of 10 µM14C-cyanate. The rest of the cyanate is either released by urine (7%) or there is specific carbamylation of haemoglobin (7.5%) (no adverse effect to animals), the rest is bound in bones (3.3%), muscles (2.1%) and other organs of the body (Cerami et al. 1973).
Dermal Dermal toxicity study with rabbits that were exposed to 33.75 mg of CN-percutaneously showed that concentrations in various organs differ (Ballantyne 1983a). The highest concentration was in the blood (310 µg/100ml). For details about concentrations in various organs seeTable 8.8.-2.
Metabolism and excretion:
Ethanedinitrile
Metabolism of ethanedinitrile Experimental data are confirming the theoretical explanation of the same mode of action as HCN, which is based on the fact that ethanedinitrile breaks down in aqueous solution into cyanide ion (CN-) and OCN-ions (Cotton and Wilkinson 1980). Ethanedinitrile due to its low log Kow (0.07) and relatively high solubility in water (2.34 g/L) is expected to get dissolved in aqueous solutions in lungs to enter the body. The rate of hydrolysis of ethanedinitrile is very fast (Ajwa 2015). Therefore, inhalation of ethanedinitrile leads to exposure to CN-(ATSDR 2006) and OCN-. The products of hydrolyses are hydrogen cyanide and cyanic acid (HOCN) (Cotton and Wilkinson 1980; Yngard et al. 2008). Both of these compounds are already included in the ADME process of hydrogen cyanide in the body – see Figure 8.8.-1 (ATSDR 2006). Thus, the metabolism and excretion described in this section (5.1.2) for cyanides and in section 5.1.1 for cyanides administred by oral route is also relevant for ethanedinitrile.
Cyanides
Metabolism and excretion of cyanides (HCN) The same way of metabolism and excretion is relevant as in part CA 5.1.1. Thiocyanate was found in the urine of non-exposed people at average concentrations of 2.16 mg/L urine for non-smokers and 3.2 mg/L urine for smokers (Chandra et al. 1980,Table 8.8.-2).
Metabolism and excretion of HOCN (NaOCN) NaOCN is metabolised in the body mainly to CO2or bounds to haemoglobin (7.5%) or other organs and tissues (see distribution part). Approximately 7% is excreted by urine and 72% is released as CO2by lungs (Cerami et al. 1973). |
Key value for chemical safety assessment
- Bioaccumulation potential:
- no bioaccumulation potential
- Absorption rate - oral (%):
- 0
- Absorption rate - dermal (%):
- 0
Additional information
Table 8.8.-1 Absorption, distribution, metabolism and excretion of cyanide
|
Tab 8.8.-2: Absorption, distribution, metabolism and excretion – other routes
|
Information on Registered Substances comes from registration dossiers which have been assigned a registration number. The assignment of a registration number does however not guarantee that the information in the dossier is correct or that the dossier is compliant with Regulation (EC) No 1907/2006 (the REACH Regulation). This information has not been reviewed or verified by the Agency or any other authority. The content is subject to change without prior notice.
Reproduction or further distribution of this information may be subject to copyright protection. Use of the information without obtaining the permission from the owner(s) of the respective information might violate the rights of the owner.
